Profile of pulmonary hypertension amongst patients seen with left heart failure in a Ghanaian hospital
Abdul-Subulr Yakubu, Alfred Doku, Francis Agyekum, Joseph Atiah Akamah
Corresponding author: Abdul-Subulr Yakubu, Department of Internal Medicine, Tamale Teaching Hospital, Tamale, Ghana
Received: 22 Jul 2022 - Accepted: 03 Jan 2023 - Published: 27 Jan 2023
Domain: Cardiology,Internal medicine
Keywords: Heart failure, pulmonary hypertension, Ghana
©Abdul-Subulr Yakubu et al. PAMJ Clinical Medicine (ISSN: 2707-2797). This is an Open Access article distributed under the terms of the Creative Commons Attribution International 4.0 License (https://creativecommons.org/licenses/by/4.0/), which permits unrestricted use, distribution, and reproduction in any medium, provided the original work is properly cited.
Cite this article: Abdul-Subulr Yakubu et al. Profile of pulmonary hypertension amongst patients seen with left heart failure in a Ghanaian hospital. PAMJ Clinical Medicine. 2023;11:25. [doi: 10.11604/pamj-cm.2023.11.25.36441]
Available online at: https://www.clinical-medicine.panafrican-med-journal.com//content/article/11/25/full
Research 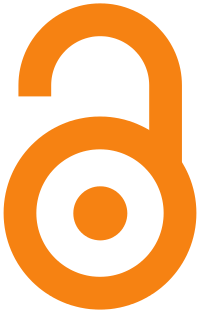
Profile of pulmonary hypertension amongst patients seen with left heart failure in a Ghanaian hospital
Profile of pulmonary hypertension amongst patients seen with left heart failure in a Ghanaian hospital
Abdul-Subulr Yakubu1,&,
Alfred Doku2,3,
Francis Agyekum2,3, Joseph Atiah Akamah3
&Corresponding author
Introduction: the development of pulmonary hypertension in left heart failure is an independent predictor of poor outcomes. We assessed the burden and predictors of pulmonary hypertension amongst patients with left heart failure presenting to the cardiology unit of Korle Bu Teaching Hospital, Ghana.
Methods: in this cross-sectional study, consecutive patients with left heart failure presenting for echocardiography were assessed for pulmonary hypertension by measuring the right ventricular outflow tract acceleration time using pulsed wave Doppler echocardiography.
Results: three hundred and fifty-five participants were recruited, of which 185 (52.1%) were male. The prevalence of pulmonary hypertension was 79.4% using a mean pulmonary artery pressure cut-off value of ≥25 mmHg. The prevalence of pulmonary hypertension was 88.4% in those with non-hypertensive heart failure compared with 71.7% in hypertensive heart failure (p<0.001). Left ventricular ejection fraction <40% (OR=6.123, 95% CI 2.674-14.019, p<0.001), New York Heart Association functional class III or IV (OR=3.324, 95% CI 1.742-6.344, p<0.001) and a systolic blood pressure <120 mmHg (OR=2.194, 95% CI 1.039-4.633, p=0.039) were independent predictors of the presence of pulmonary hypertension.
Conclusion: pulmonary hypertension is common in patients with left heart failure in our environment. Its presence was associated with poorer left ventricular systolic function, lower systolic blood pressure and more severe symptoms. Pulmonary hypertension was more common in patients with non-hypertensive heart failure. Routine assessment for pulmonary hypertension in patients with left heart failure is feasible and will aid in the risk-stratification and management of these patients.
In heart failure, the development of pulmonary hypertension (PH) marks an unfavourable clinical turning point in the natural history of the disease, which is characterized by worsening symptoms and increased morbidity and mortality [1-3]. Historically, PH has been defined as a mean pulmonary artery pressure (mPAP) of 25 mmHg or above, measured in the supine position during invasive right heart catheterization at rest [4]. Based on current evidence, the 6th World Symposium on pulmonary hypertension lowered the threshold of mPAP for the diagnosis of PH to >20 mm Hg [5]. The World Health Organization classifies PH into five subtypes [5]. Pulmonary hypertension related to left heart disease represents by far the commonest form of PH, accounting for up to 65%-80% of cases [6,7]. Few studies have addressed the issue of PH in left heart failure in Ghana. Owusu IK et al. have previously reported a mean right ventricular systolic pressure of 55.7 mmHg and a PH prevalence of 30% amongst heart failure patients attending the cardiac clinic of Komfo Anokye Teaching Hospital, Kumasi [8]. Right heart catheterization (RHC) is central to the diagnosis of PH [6]. Echocardiography, however, represents a simple and non-invasive alternative for screening for PH, especially in resource-limited settings [9]. Transthoracic echocardiography (TTE) is widely available, and a good correlation between echocardiographic estimates and directly measured pressures via RHC has been demonstrated [9]. Risk stratification is an important step in the management of patients with heart failure. Despite the prognostic significance of PH in left heart failure, it is frequently under-diagnosed [10]. We assessed the prevalence and determinants of PH amongst patients with left heart failure presenting to the cardiology unit of Korle Bu Teaching Hospital (KBTH), Ghana.
Study design and setting: the study was carried out at the cardiology unit of the Department of Medicine and Therapeutics of KBTH, a tertiary referral hospital in Ghana, from March 2020 to July 2021 using a cross-sectional study design.
Study population: the study population consisted of patients with left heart failure (with or without right heart failure) presenting for echocardiography at the cardiology unit of KBTH. Inclusion criteria were age 18 years and above and a clinical diagnosis of heart failure as defined by the Framingham criteria, as well as satisfactory images on TTE [11]. Patients with causes of elevated pulmonary pressures other than left heart disease were excluded following a thorough review of their clinical records, including relevant investigations. These included those with known chronic interstitial lung disease, chronic obstructive pulmonary disease, bronchial asthma, pulmonary arterial hypertension, connective tissue disease or congenital heart disease. Also, patients with isolated right heart failure based on clinical records, examination findings and/or absence of left ventricular (LV) systolic or diastolic dysfunction or relevant structural heart disease at echocardiography were excluded. All patients presenting for echocardiography were screened for eligibility and consecutive patients, who satisfied the inclusion criteria and did not have any exclusion criteria, were serially recruited until the sample size was attained. Applying a prevalence of PH of 70.4% amongst heart failure patients, a 5% margin of error and a 95% confidence level, a minimum sample size requirement of 321 patients was required [12,13].
Data collection: clinical data such as heart failure symptoms and their duration, current medication, comorbidities, smoking status or alcohol consumption were collected. The clinical records of the participants were reviewed to assess the clinical state of the participants and identify comorbidities. The diagnosis of diabetes mellitus and dyslipidaemia was based on a self-reported history of these conditions or concurrent treatment with anti-diabetic or lipid-lowering medication, respectively. A thorough physical examination was performed, looking for signs of heart failure and other comorbidities. Systolic blood pressure measurements ≥ 140 mmHg and/or diastolic blood pressure measurements ≥ 90 mmHg were considered diagnostic of hypertension [14]. Participants on anti-hypertensive drugs were also considered to have hypertension. Routine laboratory investigations, including the full blood count, serum electrolytes and creatinine, liver function tests, blood glucose levels and radiographs, were reviewed to assess the clinical state of the participants and identify comorbidities. The haemoglobin concentration closest to the enrolment visit (and within 3 months of enrolment) was used to define anaemia (<13.0 g/dl in men and <12.0 g/dl in women) [15]. The glomerular filtration rate was estimated using the serum creatinine value closest to enrolment (and within 3 months) with the Chronic Kidney Disease Epidemiology Collaboration (CKD-EPI) equation [16]. Each patient had a resting 12-lead electrocardiogram (ECG) recorded and interpreted using standard criteria [17,18].
All eligible participants had a standard TTE using a commercially available ultrasound machine (vivid T8 ultrasound imaging system, GE Healthcare Bio-sciences Corp, Piscataway, NJ, USA) equipped with a 3.5MHz phased array probe with the patient in the left lateral decubitus position. Standard two-dimensional (2D), Doppler and 2D-guided M mode TTE were performed in standard views according to the recommendations of the American Society of Echocardiography [19]. Cardiac chamber dimensions and wall thickness were assessed by 2D echocardiography according to the recommendations of the American Society of Echocardiography and LV ejection fraction (LVEF) calculated using the modified Simpson´s method [20]. Each echocardiogram was analyzed for pericardial abnormalities, chamber size, wall dimensions, wall motion abnormalities, ventricular function, valve morphology and function and congenital defects. For patients in atrial fibrillation, all measurements were averaged over five cardiac cycles [20,21]. To estimate the pulmonary pressure, a pulsed wave flow signal was obtained just proximal to the pulmonary valve in the parasternal short-axis view at end-expiration and the right ventricular outflow tract (RVOT) acceleration time measured from the beginning of the flow to the peak flow velocity (Figure 1) [9]. The mPAP was estimated from the formula derived by Dabestani et al. [22]: mPAP = 90 - (0.62 × RVOT acceleration time). Pulmonary hypertension was defined as a mPAP ≥ 25 mmHg. The final diagnosis of left heart failure was based on the concurrent presence of two major or one major and two minor Framingham criteria and echocardiographic evidence of LV systolic and/or diastolic dysfunction [11,23]. Minor Framingham criteria were accepted only if they could not be attributed to another medical condition. The aetiology of the left heart failure was decided on the basis of the history, physical examination, ECG, and echocardiography, as well as a review of relevant investigations. Heart failure with preserved ejection fraction (HFpEF) was defined as an LVEF of at least 50% and evidence of impaired LV diastolic function. Heart failure with reduced ejection fraction (HFrEF) was defined by the clinical diagnosis of HF and an LVEF of ≤40%, whilst those with an LVEF in the range of 41%-49% were classified as heart failure with mildly reduced ejection fraction (HFmrEF) [24].
Statistical analysis: categorical variables were presented as frequencies and percentages. Continuous variables were described with means and standard deviations for normally distributed data or median and interquartile ranges for skewed data. The normality of distribution of continuous variables was tested with the Kolmogorov-Smirnov test, as well as a visual inspection of the histograms. Chi-square analysis, or the Fisher exact test, where appropriate, was used to describe the differences between categorical variables. The independent sample t-test (or Mann-Whitney U for skewed data) and the one-way analysis of variance (or Kruskal-Wallis statistic, when appropriate) were used to compare two or more groups of continuous variables, respectively. Correlation and univariate regression analyses were conducted to examine the relationship between mPAP and various echocardiographic and clinical predictors. The variables tested included blood pressure, anthropometric indices, cardiac chamber dimensions and measures of ventricular systolic and diastolic function. Variables that showed a statistically significant relationship with the mPAP in the univariate analyses at an alpha level of 0.05 were used to construct multivariate regression models to assess for independent predictors of mPAP using a forward stepwise approach. The odds of having PH, adjusted for other variables in the model, were derived from multivariate binary logistic regression analysis. A p-value less than 0.05 was considered statistically significant. The Data obtained was entered and analyzed using the Statistical Package for the Social Sciences version 20.0 software (SPSS, IBM Corporation, Armonk, NY, USA).
Ethical considerations: the study protocol conformed to the ethical guidelines of the declaration of Helsinki on the principles for medical research involving human subjects [25]. The study protocol was approved by the KBTH Institutional Review Board (KBTH-IRB/000149/2019).
Baseline clinical and demographic data: a total of 355 participants were recruited for the study, of which 185 (52.1%) were male. The mean age of the participants was 57.4 ± 15.3 years. Systemic hypertension was the commonest comorbidity, being present in 75.1% of males and 66.5% of females. New York Heart Association (NYHA) class III or IV symptoms were reported by 63.1% of the participants. 166 (46.8%) of the participants had clinical features of isolated left ventricular failure, whilst 189 (53.2%) presented with features of biventricular failure. The median duration of heart failure symptoms at the time of recruitment was 5.0 (1.0-12.0) months. Heart failure with reduced ejection fraction was present in 49.3% of the participants, whilst 11.0% and 39.7% had HFmrEF and HFpEF, respectively. Only 54.3% of those with HFrEF were taking a renin-angiotensin system (RAS) inhibitor, whilst 38.3% took beta-blockers, and 29.1% took mineralocorticoid receptor antagonists (MRA). Table 1 summarizes the demographic and clinical information of the participants according to the presence or absence of PH.
Summary of electrocardiographic findings: the median heart rate was 96 (81-108) beats per minute. The rhythm was sinus in 87.9% of the participants. Atrial fibrillation was present in 11.3% of those with PH and 6.8% of those without PH Atrial anomalies on ECG were significantly more prevalent amongst those with PH (p<0.001). Overall, 335 (94.4%) of the participants had ECGs that were judged to be abnormal. Repolarization abnormalities and an abnormal ECG were more likely to be present in those with PH.
Summary of laboratory findings: the mean estimated glomerular filtration (eGFR) was 66.4 ± 37.4 ml/min/1.73m2 and did not differ significantly with pulmonary pressure (p=0.183). An eGFR<60 ml/min/1.73m2 was present in 23.9% of the participants. Serum sodium levels were lower in those with PH (p=0.034). The average haemoglobin level was 11.4 ± 2.4 g/dl, and 38.6% had haemoglobin levels in the anaemic range. Total bilirubin concentration was significantly higher in the presence of PH (p=0.001).
Echocardiographic characteristics and prevalence of pulmonary hypertension: using a mPAP of 25 mm Hg as the threshold for PH, the prevalence of PH was 79.4%. Pulmonary hypertension was significantly more prevalent in those with HFrEF (95.4%) compared with those with HFmrEF (74.4%) and those with HFpEF (61.0%) (p<0.001). When a mPAP of 20 mm Hg was set as the threshold for defining PH, the overall prevalence of PH rose from 79.4% to 87.3% (Figure 2). Table 2 shows the echocardiographic findings of the participants stratified according to the mPAP. The table shows that there were significant differences in most of the echocardiographic parameters between those with and without PH. Right ventricular-pulmonary artery coupling, assessed using the ratio of tricuspid annular plane systolic excursion (TAPSE) to pulmonary artery systolic pressure (PASP), was significantly worse in the group with PH.
Pulmonary hypertension and aetiology of heart failure: Table 3 summarizes the aetiology of heart failure in the study participants, as well as the prevalence of PH in each aetiologic group. Hypertensive heart disease was the commonest cause of heart failure, accounting for 53.8% of all cases. “Other” heart failure aetiologies included 2 cases of suspected tachycardiomyopathy, 2 cases of suspected myocarditis, 4 cases of hypertrophic cardiomyopathy, 3 cases of cancer therapeutics-related cardiac dysfunction, 1 case associated with thyroid dysfunction and 7 cases of left ventricular systolic or diastolic dysfunction of uncertain aetiology. The mPAP and the prevalence of PH was significantly higher in those with non-hypertensive heart failure (p<0.001). Figure 3 shows the aetiologies of heart failure in those with and without PH. The proportion of those with dilated cardiomyopathy was higher in those with PH (28.72%) than in those without PH (6.85%).
Predictors of pulmonary hypertension: multivariate linear regression analyses were performed using variables that showed a significant correlation with mPAP in the univariate analysis. The final prediction model for mPAP contained the LVEF, the left atrial volume index (LAVI), the right atrial area (RAA) and the heart rate. The model was statistically significant, F (4, 284) = 54.038, p <0 .001, and accounted for approximately 42.4% of the variance of mPAP (R2 = 0.432, adjusted R2 = 0.424). Left ventricular ejection fraction received the strongest weight in the model. A logistic regression analysis was performed to ascertain the effects of various clinical and demographic characteristics on the likelihood that the participants would have PH. Variables that showed a significant correlation at an alpha level of 0.05 in the univariate analyses were included in the multivariable analysis (Table 4). The final logistic regression model, which included LVEF <40%, systolic blood pressure (SBP) <120 mm Hg and HYHA functional class (III or IV), was statistically significant, (χ2 (3) = 81.849, p < 0.001) and explained 32.3% (Nagelkerke R2) of the variance in mPAP and correctly classified 77.7% of cases.
We assessed the prevalence and predictors of PH amongst patients with left heart failure presenting for echocardiography at the cardiology unit of KBTH. One of the main findings of the present study was the high prevalence of PH amongst patients with left heart failure, regardless of the heart failure aetiology. The LVEF, NYHA functional class (III or IV) and SBP were independent predictors of the presence of PH. Right ventricular systolic function was significantly worse in the presence of PH, and those with non-hypertensive heart failure had a significantly higher burden of PH. There is a wide variation in the reported prevalence of PH in left heart failure in sub-Saharan Africa, partly due to heterogeneity in the studies and disparity in the method of estimating pulmonary pressure and the cut-off values used [7,13,26]. In the present study, the prevalence of PH amongst patients with left heart failure was 79.4%. When a mPAP of 20 mmHg was considered as the upper limit of normal, based on recent proposals, the prevalence of PH in left heart failure rose from 79.4% to 87.3% [5,6].
The prevalence of PH increased significantly with decreasing LVEF and the presence of an LVEF < 40% was predictive of a 6-fold increased odds of the presence of PH (OR 6.123, 95% CI, 2.674-14.019, p<0.001). In clinical practice, many patients with heart failure and HFrEF are either not prescribed guideline-directed medical therapy (GDMT) for which they are eligible or are prescribed therapies at sub-target doses [27]. Older age, hypotension, lower BMI, worsening renal function and hyperkalaemia have been identified as being associated with non-use or sub-target dosing of GDMT for HFrEF [27-29]. The present study found that 54.3% of patients with HFrEF were taking a RAS inhibitor, whilst only 38.3% took beta-blockers and 29.1% took MRAs. This low rate of utilization of GDMT for heart failure has been reported across sub-Saharan Africa and may contribute to the more severe symptoms and the high prevalence of PH amongst these patients [30]. Attention should be paid to addressing the barriers to utilization of efficacious GDMT for HF and allocation of advanced therapies, where available, in order to improve outcomes [30,31].
We found that the presence of a SBP less than 120 mm Hg was associated with twice the odds of having PH (OR 2.194, 95% CI, 1.039-4.633, p=0.039). In a subgroup analysis of 242 patients with acute decompensated heart failure, Aronson et al. found that patients with PH were more likely to present with lower blood pressure (p=0.006) [32]. The relationship between SBP and mPAP amongst patients with heart failure is contrary to that found in studies in the general population without heart failure [13,33,34]. It is well known that in advanced heart failure, SBP is usually low, even in patients who were previously hypertensive [35]. This phenomenon, which has been termed “decapitated hypertension”, occurs when patients who were previously hypertensive progressively develop normal and even low blood pressure as heart failure worsens [36]. The decrease in SBP is the result of reduced pump function and a fall in cardiac output, despite the compensatory peripheral vasoconstriction [35]. Although hypertension is a well-known risk factor for incident heart failure, numerous studies have shown that higher SBP in patients with established heart failure seems to paradoxically have a protective effect on survival [37,38]. The observation that the mPAP was significantly higher in heart failure of non-hypertensive aetiology may be explained by more severe systolic dysfunction with restrictive filling in these patients.
The presence of PH was associated with significant cardiac remodelling. Pulmonary hypertension in left-sided heart failure is not only caused by the backward transmission of pressures, but also involves impairment of atrial function and inflammation [39]. In addition to left ventricular remodelling and dysfunction, an increase in left atrial size, interstitial fibrosis causing left atrial stiffness, and reduced left atrial compliance, as well as impaired left atrial contractility, contribute to the alterations in the pulmonary circuit and right heart [3]. Also, right atrial dilation commonly represents the first sign of PH in left heart failure before right ventricle (RV) dilatation and systolic dysfunction develop, ultimately leading to right heart failure [39]. The heart rate was independently associated with the calculated mPAP on multivariate linear regression analysis. Increasing heart rate is a manifestation of worsening HF with an associated increase in adrenergic activity. It is also known that the increased sympathetic activation accompanying severe heart failure also bears a close relationship with the pulmonary artery pressures [40,41]. The association of tachyarrhythmias with worsening ventricular function could also account for the observed association of heart rate and pulmonary pressure. Other contributory factors include anaemia and thyroid hyperactivity. However, blood haemoglobin concentration, as well as non-sinus rhythm, was not correlated with mPAP in the present study.
Our observation that RV systolic dysfunction was significantly more prevalent in heart failure patients with PH was not unexpected, as PH has been postulated as one of the mechanisms of RV dysfunction in left heart failure [39]. Right heart dysfunction occurs following the increased load imposed by the elevated left atrial pressure associated with diastolic dysfunction of the LV as well as atrial myopathy. The occurrence of pulmonary vascular disease and wall remodelling further exacerbates the right heart impedance through an increase in resistive load [42]. Right ventricular-pulmonary artery coupling, assessed with the TAPSE/PASP ratio, was significantly worse in the presence of PH. Patients with heart failure and RV dysfunction have been documented to show clinical and echocardiographic evidence of more advanced heart failure, such as lower LVEF, worse diastolic dysfunction, lower blood pressure and cardiac output, higher PASP, and more severe RV enlargement and tricuspid valve regurgitation [43,44].
There was no statistically significant difference in demographic and clinical parameters such as age, gender, height, body weight, body surface area or diastolic blood pressure between those with and without PH in the current study. Other authors have, however, reported that older age and greater body mass index were associated with the presence of PH in HFrEF [2]. Geographic differences in socio-demographics, cardiovascular risk factors and heart failure aetiology could contribute to the differences noted in the influence of demographics on PH prevalence. The present study had some limitations. Being hospital-based and employing a non-probability sampling technique limits the generalizability of the results. Right heart catheterization remains the gold standard for the confirmation of PH. However, it is an invasive test with a risk of complications. Therefore, for routine clinical purposes, TTE which is inexpensive and widely available with acceptable diagnostic accuracy is an appropriate initial test for PH [9,10]. Also, since the indications for echocardiography are usually symptom-driven, referral bias could have led to the recruitment of patients with more severe disease. Additionally, the determination of heart failure aetiology was based on clinical features, ECG and echocardiographic findings. Information about coronary anatomy and functional ischaemia tests were not routinely available, so it is conceivable that some participants with significant ischaemic heart disease were not identified as such.
Pulmonary hypertension is common amongst Ghanaian patients with left heart failure, occurring in over three-quarters of the participants in this study with a higher burden in those with non-hypertensive heart failure. Poor left ventricular systolic function, lower systolic blood pressure and more severe symptoms were independent predictors of the presence of PH. Routine assessment for pulmonary hypertension should be part of the echocardiographic examination of these patients to aid in risk stratification and to tailor further workup, management and follow-up. Large community-based studies are needed to better assess the outcomes of PH in patients with left heart failure in sub-Saharan Africa.
What is known about this topic
- Pulmonary hypertension is a predictor of poorer outcomes in patients with heart failure;
- Echocardiography is a useful initial screening tool for PH;
- Pulmonary hypertension related to left heart disease is the commonest form of PH.
What this study adds
- Pulmonary hypertension is common in Ghanaian patients with left heart failure over a broad range of LV systolic function;
- The burden of PH was higher in those with non-hypertensive heart failure;
- Poorer left ventricular systolic function, lower systolic blood pressure and more severe symptoms were independent predictors of the presence of pulmonary hypertension.
The authors declare no competing interest.
Abdul-Subulr Yakubu conceptualized the study, analyzed the data and wrote the initial manuscript. Alfred Doku and Joseph Atiah Akamah gave advice on the design of the study and supervised the writing of the manuscript. Francis Agyekum revised the manuscript. All authors contributed to and approved the final manuscript.
Table 1: clinical characteristics of subjects according to pulmonary pressure
Table 2: echocardiographic characteristics stratified by pulmonary pressure
Table 3: aetiology of heart failure and pulmonary hypertension (PH) prevalence in each aetiologic group
Table 4: independent predictors of pulmonary hypertension (PH)
Figure 1: pulse wave Doppler of the right ventricular outflow tract (RVOT) showing mid-systolic notching (arrows)
Figure 2: prevalence of pulmonary hypertension stratified according to LV ejection fraction (LVEF)
Figure 3: (A,B) heart failure aetiology in those with and without pulmonary hypertension; DCM, dilated cardiomyopathy, HHD, hypertensive heart disease; the category "others" include those with ischaemic heart disease, valvular heart disease and other cardiomyopathies
- Guazzi M, Ghio S, Adir Y. Pulmonary Hypertension in HFpEF and HFrEF: JACC Review Topic of the Week. J Am Coll Cardiol. 2020 Sep 1;76(9):1102-11. PubMed | Google Scholar
- Miller WL, Grill DE, Borlaug BA. Clinical features, hemodynamics, and outcomes of pulmonary hypertension due to chronic heart failure with reduced ejection fraction: Pulmonary hypertension and heart failure. JACC Hear Fail. 2013 Aug;1(4):290-9. PubMed | Google Scholar
- Rosenkranz S, Gibbs JSR, Wachter R, De Marco T, Vonk-Noordegraaf A, Vachiéry JL. Left ventricular heart failure and pulmonary hypertension. Eur Heart J. 2016 Mar 21;37(12):942-54. PubMed | Google Scholar
- Maron BA, Brittain EL, Choudhary G, Gladwin MT. Redefining pulmonary hypertension. Lancet Respir Med. 2018 Mar 1;6(3):168-70. PubMed | Google Scholar
- Simonneau G, Montani D, Celermajer DS, Denton CP, Gatzoulis MA, Krowka M et al. Haemodynamic definitions and updated clinical classification of pulmonary hypertension. Eur Respir J. 2019 Jan 24;53(1):1801913. PubMed | Google Scholar
- Al-Omary MS, Sugito S, Boyle AJ, Sverdlov AL, Collins NJ. Pulmonary Hypertension Due to Left Heart Disease: Diagnosis, Pathophysiology, and Therapy. Hypertension. 2020 Jun 1;75(6):1397-408. PubMed | Google Scholar
- Thienemann F, Dzudie A, Mocumbi AO, Blauwet L, Sani MU, Karaye KM et al. The causes, treatment, and outcome of pulmonary hypertension in Africa: Insights from the Pan African Pulmonary Hypertension Cohort (PAPUCO) Registry. Int J Cardiol. 2016;221:205-11. PubMed | Google Scholar
- Owusu IK, Adu-Boakye Y. Prevalence and Aetiology of Heart Failure in Patients Seen at a Teaching Hospital in Ghana. J Cardiovasc Dis Diagnosis. 2013 Nov 16;01(05):1-4. Google Scholar
- Parasuraman S, Walker S, Loudon BL, Gollop ND, Wilson AM, Lowery C et al. Assessment of pulmonary artery pressure by echocardiography-A comprehensive review. Int J Cardiol Hear Vasc. 2016 Sep;12:45-51. PubMed | Google Scholar
- Chung K, Strange G, Codde J, Celermajer D, Scalia GM, Playford D. Left Heart Disease and Pulmonary Hypertension: Are We Seeing the Full Picture. Heart Lung Circ. 2018 Mar 1;27(3):301-9. PubMed | Google Scholar
- McKee PA, Castelli WP, McNamara PM, Kannel WB. The Natural History of Congestive Heart Failure: The Framingham Study. N Engl J Med. 1971 Dec 23;285(26):1441-6. PubMed | Google Scholar
- Chadha VK. Sample size determination in health studies. NTI Bull. 2006;42(3&4):55-62. Google Scholar
- Amadi VN, Ajayi OE, Akintomide AO, Abiodun OO, Bamikole OJ, Balogun MO. Pulmonary hypertension in heart failure patients presenting at OAUTHC, Ile-Ife, Nigeria. Clin Med Insights Cardiol. 2016;10:187-93. PubMed | Google Scholar
- Unger T, Borghi C, Charchar F, Khan NA, Poulter NR, Prabhakaran D et al. 2020 International Society of Hypertension Global Hypertension Practice Guidelines. Hypertension. 2020 Jun 1;75(6):1334-57. PubMed | Google Scholar
- Grote Beverborg N, van Veldhuisen DJ, van der Meer P. Anemia in Heart Failure: Still Relevant? JACC Hear Fail. 2018 Mar 1;6(3):201-8. PubMed| Google Scholar
- Levey AS, Stevens LA, Schmid CH, Zhang Y, Castro AF, Feldman HI et al. A new equation to estimate glomerular filtration rate. Ann Intern Med. 2009 May 5;150(9):604-12. PubMed | Google Scholar
- Kligfield P, Gettes LS, Bailey JJ, Childers R, Deal BJ, Hancock EW et al. Recommendations for the standardization and interpretation of the electrocardiogram: part I: the electrocardiogram and its technology a scientific statement from the American Heart Association Electrocardiography and Arrhythmias Committee, Council on Clin. J Am Coll Cardiol. 2007 Mar 13;49(10):1109-27. Google Scholar
- Hancock EW, Deal BJ, Mirvis DM, Okin P, Kligfield P, Gettes LS. AHA/ACCF/HRS Recommendations for the Standardization and Interpretation of the Electrocardiogram. Part V: Electrocardiogram Changes Associated With Cardiac Chamber Hypertrophy A Scientific Statement From the American Heart Association Electrocardiography. J Am Coll Cardiol. 2009 Mar 17;53(11):992-1002. PubMed | Google Scholar
- Mitchell C, Rahko PS, Blauwet LA, Canaday B, Finstuen JA, Foster MC et al. Guidelines for Performing a Comprehensive Transthoracic Echocardiographic Examination in Adults: Recommendations from the American Society of Echocardiography. J Am Soc Echocardiogr. 2019 Jan 1;32(1):1-64. PubMed | Google Scholar
- Lang RM, Badano LP, Mor-Avi V, Afilalo J, Armstrong A, Ernande L et al. Recommendations for cardiac chamber quantification by echocardiography in adults: An update from the American society of echocardiography and the European association of cardiovascular imaging. Eur Heart J Cardiovasc Imaging. 2015 Mar 1;16(3):233-71. PubMed | Google Scholar
- Nagueh SF, Kopelen HA, Quiñones MA. Assessment of left ventricular filling pressures by Doppler in the presence of atrial fibrillation. Circulation. 1996;94(9):2138-45. PubMed | Google Scholar
- Dabestani A, Mahan G, Gardin JM, Takenaka K, Burn C, Allfie A et al. Evaluation of pulmonary artery pressure and resistance by pulsed Doppler echocardiography. Am J Cardiol. 1987 Mar 1;59(6):662-8. PubMed | Google Scholar
- Ponikowski P, Voors AA, Anker SD, Bueno H, Cleland JGF, Coats AJS et al. 2016 ESC Guidelines for the diagnosis and treatment of acute and chronic heart failure. Eur Heart J. 2016 Jul 14;37(27):2129-2200m. PubMed | Google Scholar
- Bozkurt B, Coats AJS, Tsutsui H, Abdelhamid CM, Adamopoulos S, Albert N et al. Universal definition and classification of heart failure: a report of the Heart Failure Society of America, Heart Failure Association of the European Society of Cardiology, Japanese Heart Failure Society and Writing Committee of the Universal Definition of Heart Failure: Endorsed by the Canadian Heart Failure Society, Heart Failure Association of India, Cardiac Society of Australia and New Zealand, and Chinese Heart Failure Association. Eur J Heart Fail. 2021 Mar 1;23(3):352-80. PubMed | Google Scholar
- World Medical Association. World Medical Association declaration of Helsinki: Ethical principles for medical research involving human subjects. JAMA. 2013 Nov 27;310(20):2191-4. PubMed | Google Scholar
- Kalogeropoulos AP, Siwamogsatham S, Hayek S, Li S, Deka A, Marti CN et al. Echocardiographic assessment of pulmonary artery systolic pressure and outcomes in ambulatory heart failure patients. J Am Heart Assoc. 2014 Feb 3;3(1):e000363. PubMed | Google Scholar
- Greene SJ, Tan X, Yeh YC, Bernauer M, Zaidi O, Yang M et al. Factors associated with non-use and sub-target dosing of medical therapy for heart failure with reduced ejection fraction. Heart Fail Rev. 2022 May;27(3):741-753. PubMed | Google Scholar
- Chang HY, Wang CC, Wei J, Chang CY, Chuang YC, Huang CL et al. Gap between guidelines and clinical practice in heart failure with reduced ejection fraction: Results from TSOC-HFrEF registry. J Chinese Med Assoc. 2017 Dec 1;80(12):750-7. PubMed | Google Scholar
- Chin KL, Skiba M, Tonkin A, Reid CM, Liew D, Krum H et al. The treatment gap in patients with chronic systolic heart failure: a systematic review of evidence-based prescribing in practice. Heart Fail Rev. 2016 Jul 27;21(6):675-97. PubMed | Google Scholar
- Ajayi AA, Sofowora GG, Ladipo GO. Explaining Heart Failure Hyper-mortality in Sub Saharan Africa: Global Genomic and Environmental Contribution Review. J Natl Med Assoc. 2020 Apr 1;112(2):141-57. PubMed | Google Scholar
- Lewsey SC, Breathett K. Racial and ethnic disparities in heart failure: current state and future directions. Curr Opin Cardiol. 2021 May 1;36(3):320-8. PubMed | Google Scholar
- Aronson D, Eitan A, Dragu R, Burger AJ. Relationship between reactive pulmonary hypertension and mortality in patients with acute decompensated heart failure. Circ Hear Fail. 2011 Sep;4(5):644-50. PubMed | Google Scholar
- Kushimo OA, Mbakwem AC, Ajuluchukwu JNA, Amadi CE. Clinical and echocardiographic correlates of pulmonary hypertension among heart failure patients in Lagos, south-western Nigeria. Cardiovasc J Afr. 2019 Feb 28;30(1):9-14. PubMed | Google Scholar
- Jingi AM, Noubiap JJ, Tankeu AT, Mfeukeu-Kuate L, Nkoke C, Kamdem P et al. Prevalence and determinants of pulmonary hypertension in a group of Cameroonian patients without chronic lung disease: a cross-sectional echocardiographic study. BMC Res Notes. 2017 Dec 7;10(1):571. PubMed | Google Scholar
- Messerli FH, Rimoldi SF, Bangalore S. The Transition From Hypertension to Heart Failure: Contemporary Update. JACC Hear Fail. 2017 Aug 1;5(8):543-51. PubMed | Google Scholar
- Oakley C. Diagnosis and natural history of congested (dilated) cardiomyopathies. Postgrad Med J. 1978 Jul 1;54(633):440-7. PubMed | Google Scholar
- Adamopoulos C, Zannad F, Fay R, Mebazaa A, Cohen-Solal A, Guize L et al. Ejection fraction and blood pressure are important and interactive predictors of 4-week mortality in severe acute heart failure. Eur J Heart Fail. 2007 Sep 1;9(9):935-41. PubMed | Google Scholar
- Bhatia RS, Tu JV, Lee DS, Austin PC, Fang J, Haouzi A et al. Outcome of Heart Failure with Preserved Ejection Fraction in a Population-Based Study. N Engl J Med. 2006 Oct 8;355(3):260-9. PubMed | Google Scholar
- Marra AM, Benjamin N, Cittadini A, Bossone E, Grünig E. When Pulmonary Hypertension Complicates Heart Failure. Heart Fail Clin. 2020;16(1):53-60. PubMed | Google Scholar
- Kaye DM, Lambert GW, Lefkovits J, Morris M, Jennings G, Esler MD. Neurochemical evidence of cardiac sympathetic activation and increased central nervous system norepinephrine turnover in severe congestive heart failure. J Am Coll Cardiol. 1994;23(3):570-8. PubMed | Google Scholar
- Packer M. The neurohormonal hypothesis: A theory to explain the mechanism of disease progression in heart failure. J Am Coll Cardiol. 1992 Jul 1;20(1):248-54. PubMed | Google Scholar
- Guazzi M, Naeije R. Right Heart Phenotype in Heart Failure with Preserved Ejection Fraction. Circ Hear Fail. 2021;14(4):508-19. PubMed | Google Scholar
- Sorimachi H, Omote K, Borlaug BA. Clinical Phenogroups in Heart Failure with Preserved Ejection Fraction. Heart Fail Clin. 2021;17(3):483-98. PubMed | Google Scholar
- Obokata M, Reddy YN V, Melenovsky V, Pislaru S, Borlaug BA. Deterioration in right ventricular structure and function over time in patients with heart failure and preserved ejection fraction. Eur Heart J. 2019 Feb 21;40(8):689-97. PubMed | Google Scholar
Search
This article authors
On Pubmed
On Google Scholar
Citation [Download]
Navigate this article
Similar articles in
Warning: file_get_contents(): SSL operation failed with code 1. OpenSSL Error messages: error:0A000126:SSL routines::unexpected eof while reading in /home/panafric/panafrican-med-journal.com/content/article/index_article_common.php on line 546
Warning: file_get_contents(): SSL: Success in /home/panafric/panafrican-med-journal.com/content/article/index_article_common.php on line 546